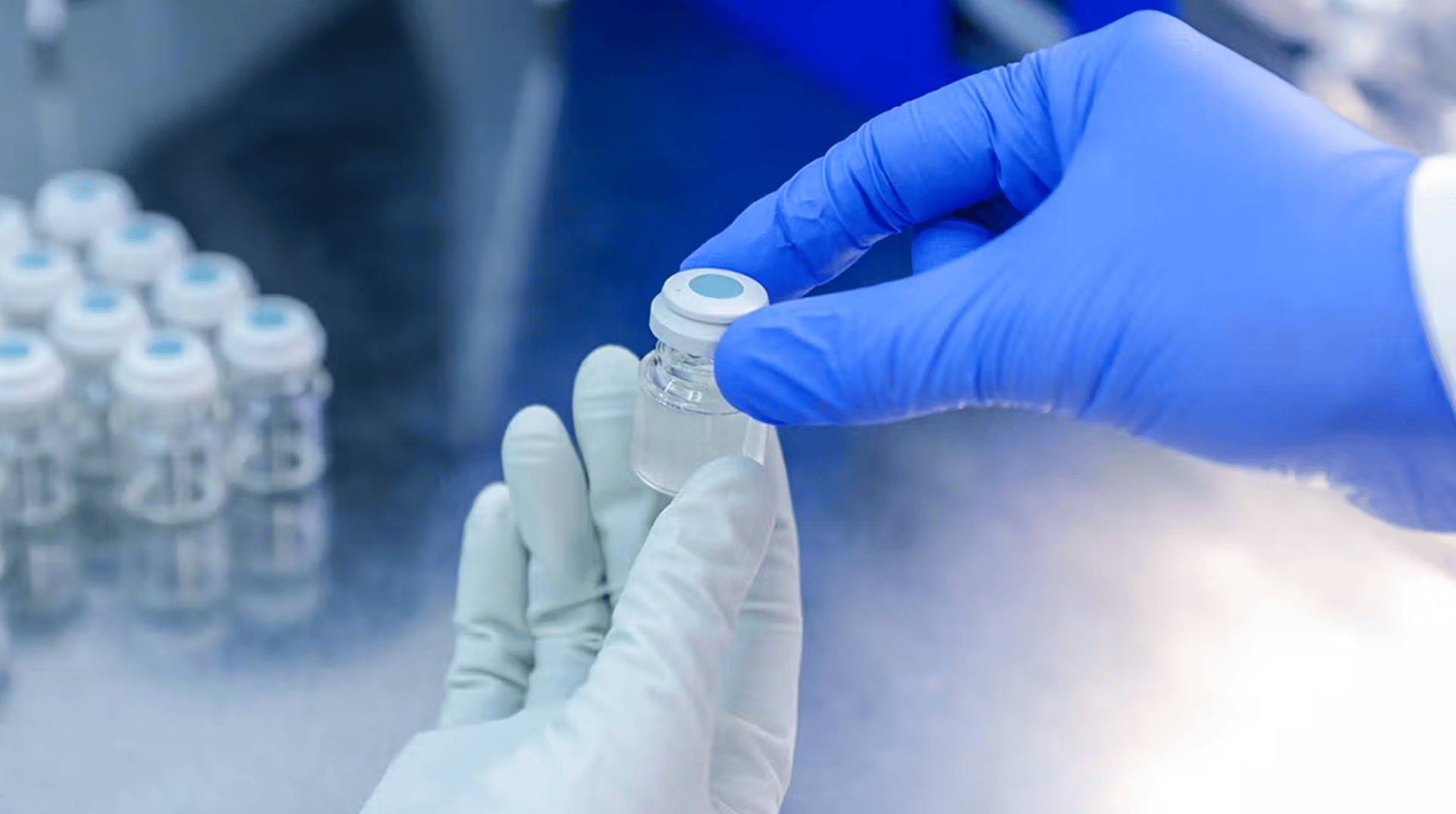
Optimizing Vaccine Lyophilization: When Can We Push Drying Limits?
BLOGS
Freeze-drying, or lyophilization, is a cornerstone for ensuring the stability of sensitive biologics like vaccines. However, the process, particularly the primary drying stage where ice is sublimated, can be lengthy and costly. Optimizing this step is crucial for efficient manufacturing.
Process development typically relies on determining critical temperatures like the glass transition (Tg') and collapse temperature (Tc). The Tc, often measured via Freeze Drying Microscopy (FDM), is traditionally considered the upper limit for the product temperature (Tp) during primary drying to prevent detrimental cake collapse. Staying several degrees below Tc is standard practice but can lead to overly conservative and long cycles.
A recent case study by Najarian et al., published in the International Journal of Pharmacy, investigated this very challenge for a vaccine formulation. Using techniques like DSC and FDM, they determined the product's critical temperatures. Initial cycles targeting Tp below the FDM-derived Tc resulted in suboptimal drying times.
Intriguingly, when the researchers applied more aggressive drying conditions where the measured product temperature reached the FDM-determined Tc, they observed no macroscopic collapse in the vials. This approach led to a remarkable ~45% reduction in primary drying time without compromising the vaccine's critical quality attributes (CQAs).
This suggests that for certain formulations, the micro-scale collapse observed in FDM might not directly translate to detrimental macroscopic collapse in the bulk product within vials. The researchers used Process Analytical Technology (PAT) like thermocouples and Manometric Temperature Measurement (MTM) to monitor the process closely. They observed specific signatures in the product temperature and resistance (Rp) profiles that correlated with the onset of unacceptable collapse, allowing them to define a more practical macro-collapse temperature (Tcm) specific to the vial drying process.
Furthermore, they successfully employed first-principles heat and mass transfer modeling to generate a primary drying design space. This modeling approach helps predict process outcomes, enabling more targeted selection of optimal parameters (shelf temperature, chamber pressure) and reducing the need for extensive experimental runs.
In essence, this study underscores:
The importance of critically evaluating the relevance of FDM-derived Tc for predicting macroscopic collapse in vials.
The value of combining direct experimental observation (temperature profiles, resistance, cake appearance) in the actual vial system to define operational limits.
The power of integrating experimental data with modeling techniques for efficient and robust lyophilization process optimization.
This approach offers a pathway to significantly shorten lyophilization cycles for vaccines and other biologics, potentially increasing manufacturing throughput and reducing costs.
Access the study: Najarian, J., Metsi-Guckel, E., Renawala, H. K., et al. (2024). Optimizing lyophilization primary drying: A vaccine case study with experimental and modeling techniques.